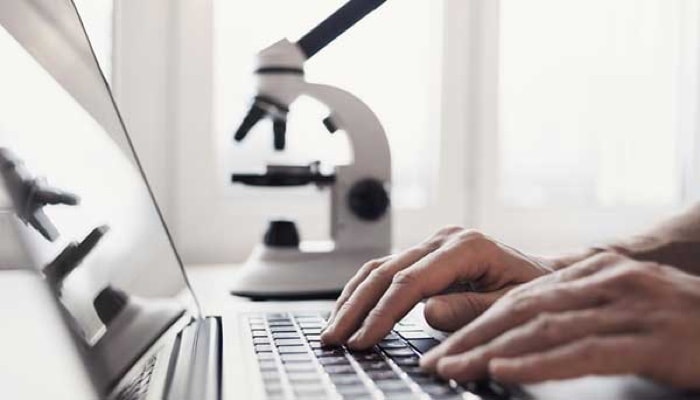
Savonia article: Microbial Contamination of the Indoor Environment – What Should We Know?
#SavoniaUAS
According to the EPA (2021), Indoor Air Quality (IAQ) refers to the air quality within and around buildings and structures, especially as it relates to the health and comfort of building occupants. The impact of IAQ on occupants’ health, comfort, satisfaction, and productivity has been one of the focuses of architecture, occupational and public health research since individuals spend about 90% of their time in indoor environments (Megahed and Ghoneim, 2021).
The adverse health effects from indoor air pollutants may be experienced soon after exposure or, possibly, years later. Therefore, understanding pollutant sources in the indoor environment and their control can help reduce the risks of indoor health concerns.
Growth and aerosolization of microbes
Building materials play a major role in the growth of microbe and their subsequent presence in the indoor environment. Pollutants released into the indoor air are usually aerosol mass consisting of non-biological particles, gaseous components, volatile organic compounds (VOCs) and bioaerosols (Fig. 1). Bioaerosols include viable or non-viable bacteria and fungi, viruses, pollens and allergens together with several secondary metabolites such as mycotoxins, β (1,3)-glucans and endotoxins (Ghosh et al., 2022). Fungal spores and their fragments are considered the most abundant fraction of the bioaerosols mass due to the abundant substrates available to them.
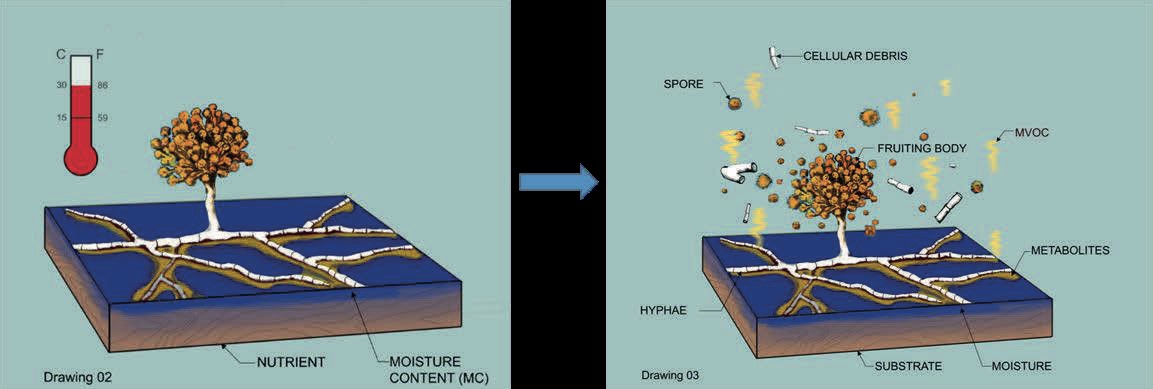
The amount of growth on a particular material depends on the material’s characteristics, for example, chemical composition, nutritional content and porosity, the fungal species, temperature and the amount of moisture available in the material (Verdier, 2014). While the chemical composition and nutritional content determine the susceptibility or resistance of a building material to fungal growth, the presence of dust increases all materials susceptibility because of its rich nutrient content (Mensah-Attipoe et al., 2015). Therefore, to eliminate or reduce fungal growth, the generation and accumulation of dust on materials surfaces should be minimized.
After the growth is aerosolization. This occurs when the detachment forces exceed the binding forces. Driving factors include airflow over the growth surface, age of the growth, relative humidity on the growth, fungal species, and type of building material (Li et al., 2022).
Health effects of fungal exposure
The adverse health effects associated with fungal exposures can be caused by the fungi, fungal mycotoxins and cell wall components or metabolically produced compounds (Nevalainen et al., 2015). The health effects include 1) infections, caused by the viable cells (Nevalainen et al., 2015); 2) allergic reactions, caused by both viable and non-viable cells and components of the cell wall of the fungi if they carry antigens (Akar-Ghibril & Phipatanakul, 2020) and 3) toxic response to the mycotoxins produced by the fungi (Norback and Cai, 2019). Non-specific symptoms such as eye, nose and throat irritation and fatigue have often been found in connection with building related problems (Fu et al., 2021).
Measurement of fungal growth on building materials
Contamination of the indoor environment as a result of fungal growth, creates a source of spores, fungal fragments, and other products that may become airborne. Measurements of airborne fungi are often used to detect such contamination. However, not all active fungal growths release spores continuously due to physiological and environmental factors, therefore, fungal measurement should be done on the material surfaces.
Measurement of the growth should be done in such a way that all fungal particles and components are assessed. Different assay methods are required since no one method can detect fungal presence. While some methods detect only viable particle, other methods detect the non-viable components and toxins. The use of different methods is necessary because of the varying growth dynamics observed for different fungal species over time (Mensah-Attipoe et al., 2016a). Each method gives different perspectives, and this is mainly dependent on the physiological state of the cells, especially the balance between growth and death.
Fungal growth can be measured using cell quantification or biomass estimation. The cultivation method remains the obvious choice for isolation, identification, and estimation of fungal growth on building materials. However, it only accounts for culturable fungi and underestimates the total fungal concentration. To adequately assess total fungal concentration, counting methods for example, the CAMNEA method (Collection of Airborne Micro-organisms on Nucleopore filters, Estimation and Analysis), which allows for the measurement of both culturable and unculturable spores is employed. Also, PCR-based methods which target the DNA of both culturable and unculturable cells can be used (Mensah-Attipoe et al., 2016a).
An alternative to fungal cell quantification is total fungal biomass estimation, which includes the use of chemical markers, e.g. ergosterol (Gessner, 2020), (1,3)-beta-d-glucan (Choi et al., 2014) and the enzyme beta-N-acetylhexosaminidase (NAHA) (Rylander, 2015). These chemical markers are fungal specific and are found in both viable and non-viable cells, therefore are good surrogates for estimating fungal biomass.
The fungal particles released into the indoor air can also be measured. These are the spores and fragments. Depending on the type of species, example, Aspergillus and Penicillium, large numbers of spores are released while for species like Cladosporium, very few spores are released (Mensah-Attipoe et al., 2016b). Fragments are usually from both biological and non-biological origin. Fragments of biological origin arise from the fungal growth itself while the non-biological particles arise from biodegradation of building material. Previous studies have verified biological origin with antigen characterization (Gorny et al., 2002). However, recent studies have attempted to differentiate the origin of the fragments using spectroscopic and elemental analysis (Mensah-Attipoe et al., 2016b).
Understanding the factors and processes of indoor contamination from building materials is very important to everyone. Hence, continuous monitoring and assessment of bioaerosol are of utmost importance for both identifying risks of exposure and establishing required threshold limits since it is evident that bioaerosols do have adverse health impact on humans (Ghosh et al., 2022). Along with assessment, appropriate measures are also necessary to control bioaerosols.
What should we do?
There is the need to establish ‘an indoor air group’ for public buildings and housing companies to help monitor structural changes and conditions that promote fungal growth, detect them early and mitigate the problems. For example, assessing moisture damage and ensuring they are repaired immediately, and ensuring the ventilation systems undergo necessary service and renovation before poor indoor air quality leads to complaints and symptoms. Also, there is the need to develop guidelines for the management and communications related to exposure conditions and characterization of related health effects.
Author:
Jacob Mensah-Attipoe, Degree Student in Nursing, School of Health Care, Savonia UAS. TNE20SP.
Supervisor: Leena Koponen, Senior Lecturer, International Bachelor’s Degree Programmes. International Business Unit. Savonia UAS.
References:
Akar-Ghibril, N., & Phipatanakul, W. 2020. The indoor environment and childhood asthma. Current allergy and asthma reports, 20(9), 1-17.
Choi, H., Byrne, S., Larsen, L. S., Sigsgaard, T., Thorne, P. S., Larsson, L., … & Bornehag, C. G. 2014. Residential culturable fungi,(1‐3, 1‐6)‐β‐d‐glucan, and ergosterol concentrations in dust are not associated with asthma, rhinitis, or eczema diagnoses in children. Indoor air, 24(2), 158-170.
Fu, X., Norbäck, D., Yuan, Q., Li, Y., Zhu, X., Hashim, J. H., … & Sun, Y. 2021. Association between indoor microbiome exposure and sick building syndrome (SBS) in junior high schools of Johor Bahru, Malaysia. Science of the Total Environment, 753, 141904.
Gessner, M. O. 2020. Ergosterol as a measure of fungal biomass. In Methods to study litter decomposition (pp. 247-255). Springer, Cham.
Ghosh, B., Das, A., Lal, H. 2022. Bioaerosol and Its Impact on Human Health. In: Sonwani, S., Shukla, A. (eds) Airborne Particulate Matter. Springer, Singapore.
Górny, R. L., Reponen, T., Willeke, K., Schmechel, D., Robine, E., Boissier, M., & Grinshpun, S. A. 2002. Fungal fragments as indoor air biocontaminants. Applied and environmental microbiology, 68(7), 3522-3531.
https://www.epa.gov/indoor-air-quality-iaq (accessed 03062022)
Li, X., Liu, D., & Yao, J. 2022. Aerosolization of fungal spores in indoor environments. Science of The Total Environment, 153003.
Megahed, N. A., & Ghoneim, E. M. 2021. Indoor Air Quality: Rethinking rules of building design strategies in post-pandemic architecture. Environmental Research, 193, 110471.
Mensah-Attipoe, J., Reponen, T., Veijalainen, A. M., Rintala, H., Täubel, M., Rantakokko, P., … & Pasanen, P. 2016a. Comparison of methods for assessing temporal variation of growth of fungi on building materials. Microbiology, 162(11), 1895-1903.
Mensah-Attipoe, J., Saari, S., Veijalainen, A. M., Pasanen, P., Keskinen, J., Leskinen, J. T., & Reponen, T. 2016b. Release and characteristics of fungal fragments in various conditions. Science of The Total Environment, 547, 234-243.
Mensah‐Attipoe, J., Reponen, T., Salmela, A., Veijalainen, A. M., & Pasanen, P. 2015. Susceptibility of green and conventional building materials to microbial growth. Indoor Air, 25(3), 273-284.
Morse, R., & Acker, D. 2009. Indoor air quality and mold prevention of the building envelope. National Institute of Building Sciences, 1, 2009.
Nevalainen, A., Täubel, M., & Hyvärinen, A. 2015. Indoor fungi: companions and contaminants. Indoor air, 25(2), 125-156.
Norbäck, D., Cai, GH. 2020. Microbial Agents in the Indoor Environment: Associations with Health. In: Kishi, R., Norbäck, D., Araki, A. (eds) Indoor Environmental Quality and Health Risk toward Healthier Environment for All. Current Topics in Environmental Health and Preventive Medicine. Springer, Singapore.
Rylander, R. 2015. β-N-Acetylhexosaminidase (NAHA) as a marker of fungal cell biomass–storage stability and relation to β-glucan. Int J Environ Monit Anal, 3(4), 205-209.
Verdier, T., Coutand, M., Bertron, A., & Roques, C. 2014. A review of indoor microbial growth across building materials and sampling and analysis methods. Building and environment, 80, 136-149.